We have demonstrated that oral and nasal immunization combined with an appropriate antigen delivery system and adjuvant can induce antigen-specific mucosal immune responses (e.g., secretory IgA), which are difficult to induce by systemic immunization. Further, we showed the essential foundation showing that mucosal immunization can induce antigen-specific systemic immunity (e.g., serum IgG) in the same way as injectable systemic immunization (1-3). Thus, mucosal immunization can provoke effective antigen-specific immune responses in the mucosal and systemic compartments as the two layers of defense system Based on this concept, we have led innovative research toward the development of mucosal vaccines from 2003. The studies are expected to develop a next-generation of oral and nasal vaccines against infectious diseases by fully using the unique background of the intestinal and respiratory immune systems (4, 5)
Nasal vaccines against respiratory infectious diseases
The novel coronavirus disease (COVID-19) that emerged in 2019 is an infectious disease caused by SARS coronavirus 2 (SARS-CoV-2) that infects humans, causing symptoms such as fever, cough, sore throat, and fatigue. In some cases, it can cause life-threatening serious symptoms such as pneumonia, dyspnea, and respiratory failure. Confusion, coma, loss of consciousness, inflammation of the brain, and effects on the heart and blood vessels have also been reported in some patients. Influenza is characterized by chills, high fever, headache, general malaise, and muscle pain, followed by respiratory tract inflammation and gastrointestinal symptoms, and depending on the case, serious symptoms such as pneumonia, respiratory failure, encephalopathy, etc., may be life-threatening.
Humankind has so far experienced the strain of medical institutions and the stoppage of social functions due to the pandemic influenza and COVID-19. In collaboration with Shionogi & Co., Ltd., which is working to free us from the threat of these respiratory infections, we are promoting research and development of intranasal vaccines against these diseases.
Cationic nanogel nasal vaccine
We have developed a nasal vaccine delivery system based on a cationic cholesterol-substituted pullulan, a hydrophobic polymer with protein internalization capability in collaboration with material engineering field (6). Because the mucosal surface is negatively charged, a cationic nanogel (cCHP) nasal vaccine improves mucosal adhesion by its cationic property. Indeed, it has been confirmed that the cCHP encapsulating vaccine antigen effectively adhere to the nasal mucosal epithelial layer including a respiratory M cells or nasopharyngeal-associated tissue (NALT) with classical M cells after nasal administration. The antigens are taken up by the epithelial cells by endocytosis, released from the cCHP nanogel, passed through the basement membrane by exocytosis, and then delivered to dendritic cells that accumulate below the nasal mucosa. The antigen-specific immune responses were effectively induced through the respiratory mucosal immune system (6-10).
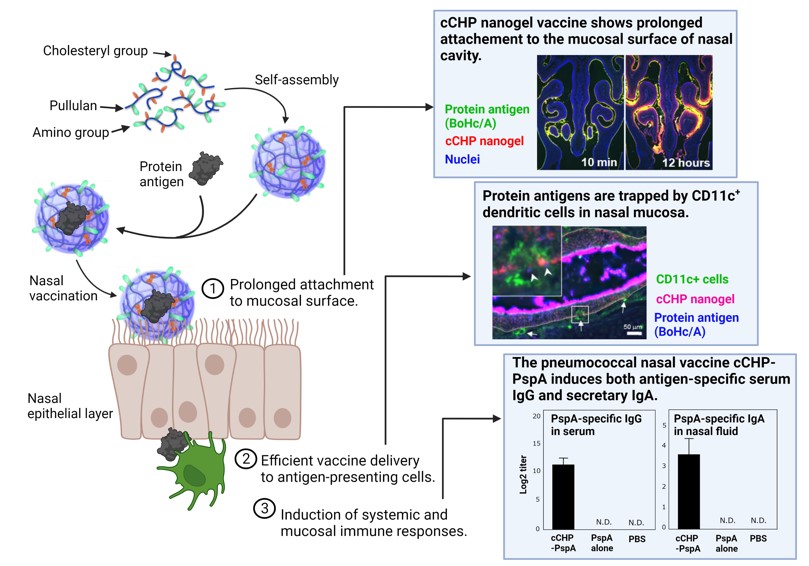
Next, we are developing a cCHP-based nasal vaccine against pneumonia. The current injectable serotype-specific vaccines target only endemic strains of S. pneumoniae, which consists of 100 serotypes, however, the spread of S. pneumoniae other than endemic strains due to serotype substitution has become a problem in clinical practice. Based on the collaborative research with the University of Alabama at Birmingham (UAB) to induce mucosal immunity using PspA, a common protein antigen of S. pneumoniae (11-14), we demonstrated that a cCHP-based pneumococcal nasal vaccine (cCHP-PspA) containing PspA effectively induced antigen-specific IgA antibodies in the respiratory mucosa to prevent S. pneumoniae invasion (15). We also confirmed that the antigen-specific IgG antibody that clear S. pneumoniae through complement pathway is induced in the lower respiratory tract. In addition, we demonstrated the safety and efficacy of the cCHP-PspA by using a non-human primate model (16, 17). We have developed a PET/MRI system in collaboration with Hamamatsu Photonics K.K. and proved the safety of the cCHP-based nasal vaccine by analyzing the vaccine deposition in the central nervous system including the brain and olfactory bulbs in primate model (16, 17). Furthermore, a trivalent cCHP-PspA, a combination of three PspA chimeric antigens with cCHP, was shown to have broad defense against different serotypes of S. pneumoniae and its efficacy in preventing pneumonia was confirmed in non-human primate model (18). In addition to Streptococcus pneumoniae, we have successfully developed a nasal vaccine for otitis media using a P6 antigen of Haemophilus influenzae, a known cause of otitis media (cCHP-P6) (19). The cCHP-based nasal vaccine development is also underway for other respiratory infections (e.g., RSV, Influenza, SARS-CoV-2). Based on this technology, a university-launched venture company, HanaVax, has been established and conducted industry-academia joint research toward the realization of nasal vaccines.
MucoRice, a rice-based oral vaccine
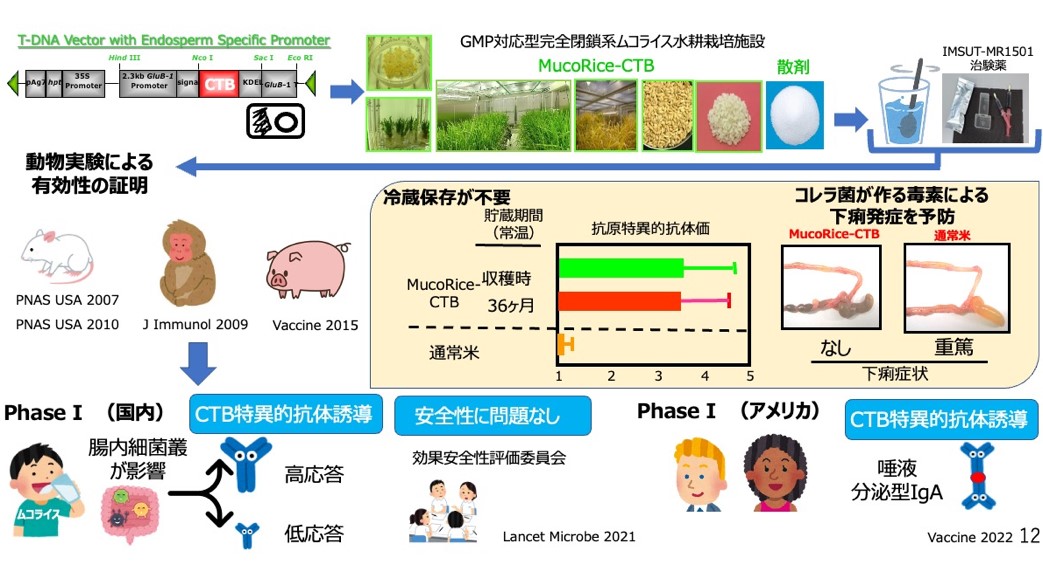
The MucoRice system is a technology that expresses, produces and stores vaccine antigens in rice seeds, enabling stable and storable oral vaccines at room temperature (20). MucoRice-CTB is a rice-based oral vaccine for cholera and traveler's diarrhea in which the non-toxic B-chain (CTB) antigen of cholera toxin (CT) is expressed on rice. MucoRice-CTB is an oral rice vaccine for cholera and traveler's diarrhea, in which the vaccine antigen is expressed in rice endosperm cells and is established as a technology for stable protein production and storage at room temperature(20, 21). MucoRice-CTB has been shown to induce antigen-specific IgG and IgA antibodies capable of neutralizing toxins as an oral vaccine in small laboratory animals (mice), large laboratory animals (pigs) and primates (20-25). Importantly, it was directly demonstrated that CTB was not effective in suppressing diarrhea despite the induction of antigen-specific serum IgG antibodies when CTB was administered as an injectable vaccine, and that CTB-specific secretory IgA induced by oral immunization with CTB-expressing rice played a critical role in suppressing diarrhea. This was demonstrated using genetically modified mice (poly Ig receptor KO mice) that are unable to produce secretory IgA and induce it on mucosal surfaces (21). In order to enable oral administration of MucoRice-CTB to humans, we succeeded in producing marker-free MucoRice-CTB51A, which does not contain the hygromycin resistance gene, a selection marker used to create transgenic rice plants (26), and established a seed bank (27, 28). In the 51A strain, a T-DNA vector containing a vaccine cassette was inserted into the rice genome in tandem on rice chromosome 3 and one copy on rice chromosome 12, and the complete transgene sequence was determined (26).
Proteomic and histological analyses were performed to evaluate the safety of the 51A strain as a genetically modified rice, and the expression level of the a amylase/trypsin inhibitor-like protein family was significantly reduced in MucoRice-CTB. We found that the 51A strain was a hypoallergenic rice strain with significantly reduced expression of the α-amylase/trypsin inhibitor-like protein family, which is a major allergen in rice (29, 30). A fully enclosed GMP facility for active pharmaceutical ingredients was established at the Institute of Medical Science, University of Tokyo, and MuocRice-CTB oral cholera vaccine was produced as an investigational drug under GMP (31). After conducting nonclinical toxicity studies, we conducted a Phase I clinical trial (Phase I) in healthy Japanese male subjects as a first-in-human, randomized, placebo-controlled, double-blind, four-treatment, dose-escalation study (1, 3, 6 g) at 2-week intervals at the University of Tokyo Medical Research Institute Hospital. Safety, tolerability and immunogenicity were confirmed in humans (32). No serious adverse events were observed in this study, and the positive rate of antigen-specific antibody induction (e.g., IgG and IgA antibodies in serum) increased with increasing dose (1 g dose: 40%, 3 g dose: 55%, 6 g dose: 75%), demonstrating immunogenicity as an oral vaccine in humans. Based on these results, we conducted a Phase IB clinical trial (Phase IB) of the 6g dose only in healthy American men and women under the US FDA Pre-IND and IND clinical trial application as the AMED CiCLE project and confirmed the safety and tolerability of the 6 g dose in the US and immunogenicity in a racially diverse group. Immunogenicity of the 6 g dose confirmed the antigen-specific secretory IgA-inducing effect of the vaccine in saliva (33).
Establishing treatment for enteric infections with oral antibodies
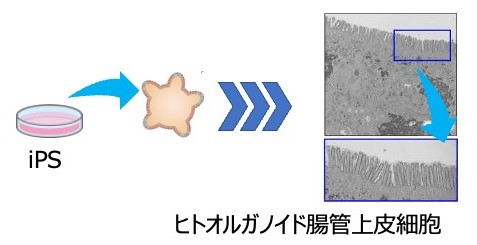
Diarrhea caused by rotavirus and norovirus infection is one of the most life-threatening diseases in children and immunocompromised patients in developing countries. We have developed a novel prophylactic and therapeutic approach to rotavirus disease using recombinant rice (MucoRice-ARP1) expressing a rotavirus-specific neutralizing llama single-chain antibody variable domain (VHH). The VHH fragment of the llama heavy chain is a small protein with a molecular weight of 12 KDa that is stable to heat and acid, making VHH an attractive and useful antibody for oral immunotherapy and prevention of enteric infections. ARP1 is one of the universal antibodies against rotavirus VP6 antigen. ARP1 in rice seeds was highly expressed (0. 85% of rice weight) due to the characteristic RNAi system of MucoRice, which suppresses the production of endogenous rice storage proteins (34). Oral administration of MucoRice-ARP1 significantly suppressed rotavirus-induced diarrhea in both healthy and immunodeficient mice. MucoRice-ARP1 was stable even after heat treatment at 94℃ for 30 minutes (34). Using this technology, we generated VHH (7C6 and 1E4) and its heterodimer antibodies (7C6-1E4) against both norovirus type GII.4, which is currently endemic worldwide, and GII.17, which is endemic in Southeast Asia including Japan (35). We have also generated a recombinant rice, MucoRice-VHH (7C6-1E4), which expresses these heterodimeric antibodies (35). MucoRice-7C6-1E4 was confirmed to neutralize both GII.4 and GII.17 noroviruses in a culture system using intestinal epithelial cells derived from human iPS cell organoids, and also showed heat resistance when treated at 90℃ for 30 minutes (36, 37).
Development of cationic nanogels and novel delivery carriers for mucosal vaccines
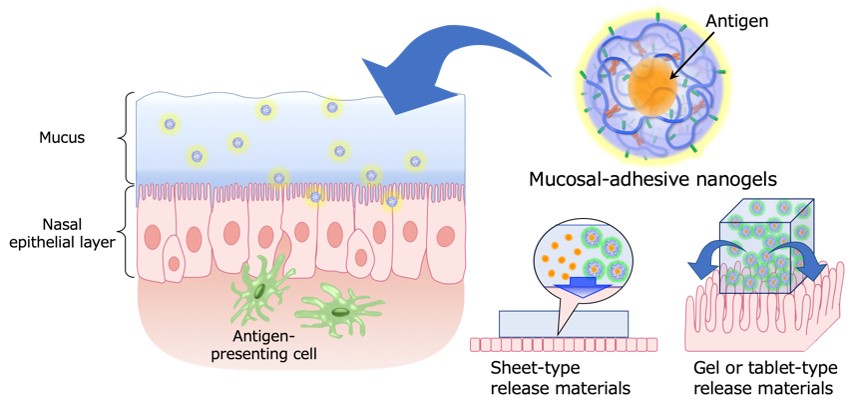
To induce antigen-specific mucosal immune responses in mucosal vaccines, it is necessary to deliver the antigen to antigen-presenting cells (dendritic cells and macrophages) that are located under the mucosal epithelial layer. Transmucosal delivery of vaccine antigens alone is often low in efficiency because of barriers such as mucus and degradative enzymes on the mucosal surface, and it is necessary to develop a new method of delivering vaccine antigens to the mucosal epithelium with protecting them. The cationic nanogels used in the above-mentioned 'cationic nanogel nasal vaccine' can hold vaccine antigens inside their nano-sized gel particles and allow the vaccine antigen to be retained on the surface of the nasal mucosa, resulting in sustained release and delivery of the antigen and antigen/nanogel complexes (6-9). Based on our previous findings, we are designing and developing cationic nanogels and novel delivery carriers to improve their functions, such as affinity with the mucosal surface layer and permeability through the mucosal epithelium. In addition, as a new administration platform for mucosal vaccines, we focus on the development of mucosal vaccine-retaining and controlled-release functional materials that can be 'pasted' and 'swallowed' from the viewpoint of polymer chemistry, and the construction of a non-invasive vaccine administration system based on these materials.
Basic research - elucidation of the mucosal immune system
Since the latter half of the 1970s, research has been conducted at the University of Alabama at Birmingham (UAB) to elucidate the uniqueness of the mucosal immune system in the gastrointestinal tract that originates from the oral cavity, the existence of which was not even well known. He has promoted research on mucosal immunity and contributed to the formation of its academic foundation. The results are connected to the theoretical and technical foundations for the development of mucosal vaccines.
Define roles of the mucosal inductive tissues in the intestinal tract.
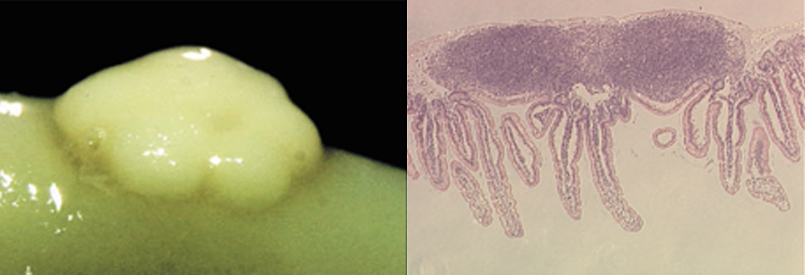
Based on the hypothesis that the Peyer's patches are the site for the induction of antigen-specific immune responses, we developed the Peyer's patches enzyme-treated cell isolation method, which is now widely used, and proved the existence of antigen-presenting cells in these tissues (38). Furthermore, we showed the presence of Th cells that selectively induce and regulate IgA responses (39, 40), which were subsequently classified as Th2-type cells. We also demonstrated that among the B cell population in the Peyer's patches, the microbiota-dependent CD11+ IgA committed B cell population can produce high levels of antigen-specific IgA antibodies (41). These results demonstrated that the Peyer's patches are the site for the induction of antigen-specific immune responses in the mucosal immune system, and provided a theoretical basis for the mechanism by which oral immunization can induce mucosal immunity that cannot be induced by injection of antigens. Furthermore, we have elucidated the cellular and molecular mechanisms of IgA antibody production, which plays a central role in mucosal immunity, and its control and regulation (39, 42-46). Furthermore, with regard to tolerance, especially oral tolerance, which is essential for the maintenance of immune homeostasis, we have devoted our efforts to elucidating its mechanism and have shown the important role of mucosal T cells and Peyer's patches (47-51).
Elucidation of the antigen uptake at the mucosal surfaces
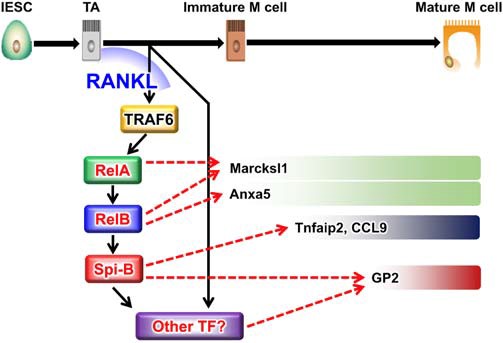
Specialized epithelial cells that are responsible for antigen uptake from the luminal side are called M cells, which reflects the uniqueness of the mucosal immune system. We succeeded to generate NKM16-2-4 monoclonal antibody which recognizes a glycosylated antigen on the M cell membrane and subsequently identified GP-2 as the M cell-specific surface antigen (3, 52, 53). In addition, we have contributed to the discovery of Spi-B and Aif1 (54, 55), a group of transcription factors that regulate the development and antigen uptake capacity of M cells. Furthermore, we reported that M cells, which play a central role in the antigen uptake mechanism in the mucosa, also exist in the villi of the epithelium, overturning the conventional theory that they exist only in the epithelial cell layer of Peyer's patches, and named them "villus M cells" (56), strongly suggesting the diversity of the antigen uptake mechanism at the mucosal surface. These results have led to the establishment of a strategic basis for the induction of antigen-specific immune responses through the gastrointestinal mucosa by oral immunization.
Regulation of Mucosal Immunity by the Gut Microbiota
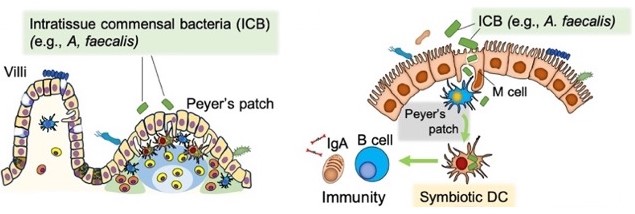
The impact of gut commensal bacteria on immune mechanisms has been a subject of interest, and as early as 1980, it was reported using germ-free animals that certain bacterial groups (e.g., Escherichia coli ) or their derived molecules (e.g., LPS) are directly involved in the development and regulation of the gut-associated immune system centered around Peyer's patches (57). As part of the lineage studies, Alcaligenes species, which are a constituent of the commensal microbiota, were discovered to establish a symbiotic environment within Peyer's patches, pioneering the concept of "tissue-resident symbiosis" (58). Subsequently, through collaborative research, it was revealed that innate lymphoid cells type 3 (ILC3) and dendritic cells are involved in the establishment of tissue-resident symbiotic environments (59, 60). More recently, it has been elucidated that LPS derived from the same bacteria plays a role in the symbiosis within dendritic cells in Peyer's patches and in the maturation of mucosal immune mechanisms. Structure analysis revealed that hexa-acylated lipid A acts as a non-inflammatory mucosal immune-activating agonist for Toll-like receptor 4 (TLR-4), and it is involved in IgA induction (61, 62). Through collaborative research, the efficacy of synthetic analogs as mucosal adjuvants has also been confirmed (63), and research and development of next-generation gut microbiota-derived non-inflammatory adjuvants are being pursued.
Proposed Concept of the Intestinal Multi-ecosystem
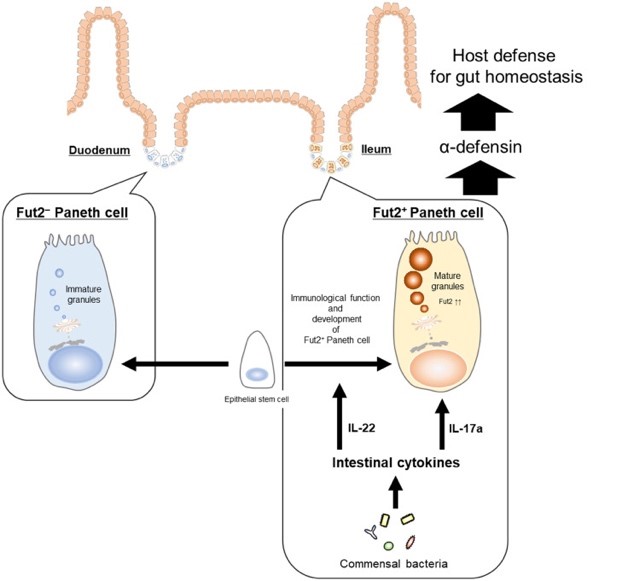
Regarding the attention given to sugar chain modifications in the intestinal environment, it has been revealed that gut commensal bacteria and innate lymphoid cells type 3 (ILC3) integrally control epithelial cell sugar chain modifications through the cytokine IL-22, thereby contributing to the prevention of microbial invasion and establishment of a symbiotic environment (64-66). The existence of mechanisms involving immune cell regulation by mesenchymal cells underlying the epithelial cells (e.g., ILCs and mast cells) and the regulation of barrier function by epithelial cells has also been demonstrated (67, 68). These findings highlight the importance of the coordinated network formed by host constituents (epithelial-mesenchymal-immune systems) and the gut microbiota in the functional mucosal epithelial barrier mechanism. This network has been proposed as the "intestinal multi-ecosystem" (69). Furthermore, it has been elucidated that Paneth cells, which are antimicrobial peptide-producing cells residing in the intestinal crypts and contribute to this network, consist of two subsets distinguished by their sugar chain modifications. Particularly, Fut2+ Paneth cells have been shown to play a crucial role in maintaining intestinal homeostasis and infection control, leading to an understanding of the role of Paneth cells in the intestinal multi-ecosystem (70).
Elucidation of respiratory and ocular mucosal immune system
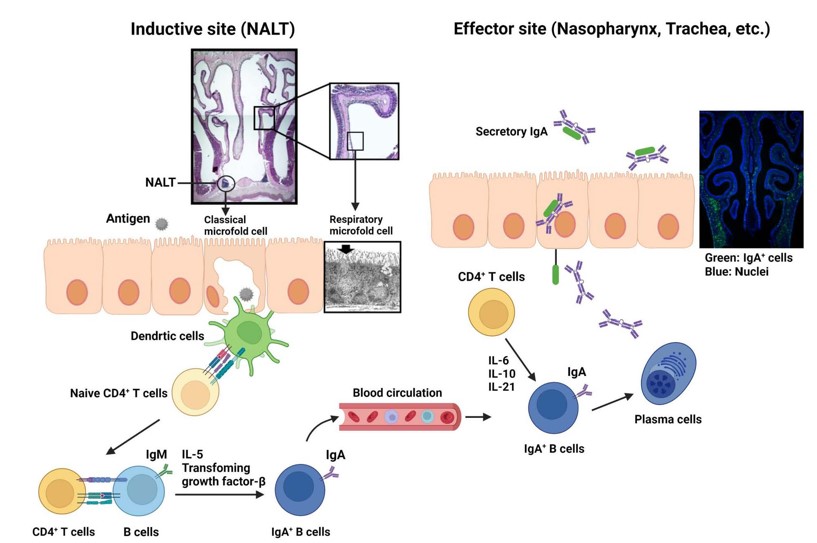
We also made progress in elucidating the respiratory and ocular mucosal immune system and identified the guiding cells and molecular mechanisms involved in the development of nasopharynx associated lymphoid tissue (NALT), that is distinct from other secondary immunocompetent lymphoid tissue formation represented by Peyer's patches and peripheral lymph nodes (49, 50). In addition, "respiratory microfold cells (M cells)," which correspond to M cells in gut, were identified in the nasal mucosa (51). In the ocular area, we identified tear duct-associated lymphoid tissue (TALT) and revealed the tissue development mechanisms (52). Through these studies, we understand that NALT and TALT are sites for the induction of antigen-specific immune responses (50,52) and these findings could be the basis for the development of nasal and eye drop vaccines.
Tissue Specificity and Organ Crosstalk in Immune Regulation
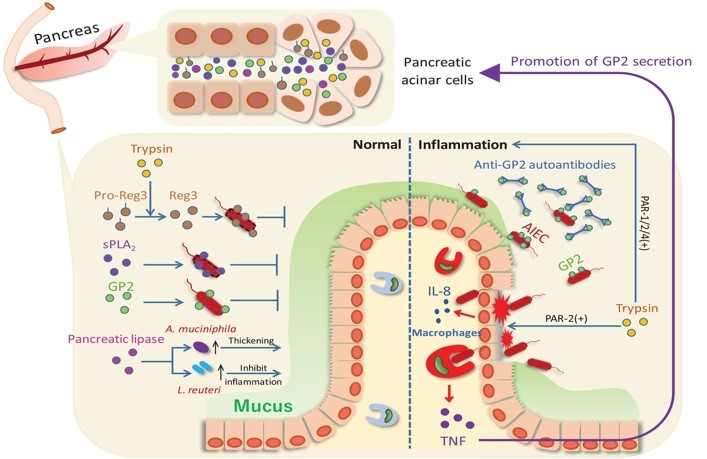
From the perspective of body surface, we have revealed the tissue specificity in the control of mast cells mediated by ATP and its receptor P2X7, produced in the intestines and skin. In the intestines, the ATP-P2X7 cascade is involved in inflammation response initiation through mast cells, while in the skin, excessive response inhibition is mediated by the inhibition of the ATP-P2X7 cascade through the retinoic acid-degrading enzyme cyp26B1 (67, 71).
Recently, we have demonstrated the existence of mucosal homeostasis and inflammation control mechanisms through pancreatic-intestinal crosstalk mediated by the pancreatic glycoprotein GP2 (72). These findings hold the potential to contribute to the development of novel therapeutics targeting tissue-specific and organ crosstalk immune regulation. These series of fundamental research achievements are paving the way for strategic development based on the uniqueness of mucosal immune mechanisms, particularly in the digestive and respiratory systems, towards the development of mucosal vaccines.
<References>
- Kurono Y, Yamamoto M, Fujihashi K, Kodama S, Suzuki M, Mogi G, McGhee JR, Kiyono H. Nasal immunization induces Haemophilus influenzae-specific Th1 and Th2 responses with mucosal IgA and systemic IgG antibodies for protective immunity. J Infect Dis. 1999;180(1):122-132.
- Kweon MN, Yamamoto M, Watanabe F, Tamura S, Van Ginkel FW, Miyauchi A, Takagi H, Takeda Y, Hamabata T, Fujihashi K, McGhee JR, Kiyono H. A nontoxic chimeric enterotoxin adjuvant induces protective immunity in both mucosal and systemic compartments with reduced IgE antibodies. J Infect Dis. 2002;186(9):1261-1269.
- Nochi T, Yuki Y, Matsumura A, Mejima M, Terahara K, Kim DY, Fukuyama S, Iwatsuki-Horimoto K, Kawaoka Y, Kohda T, Kozaki S, Igarashi O, Kiyono H. A novel M cell-specific carbohydrate-targeted mucosal vaccine effectively induces antigen-specific immune responses. J Exp Med. 2007;204(12):2789-2796.
- Azegami T, Yuki Y, Kiyono H. Challenges in mucosal vaccines for the control of infectious diseases. Int Immunol. 2014;26(9):517-528.
- Kiyono H, Yuki Y, Nakahashi-Ouchida R, Fujihashi K. Mucosal vaccines: wisdom from now and then. Int Immunol. 2021;33(12):767-774.
- Nochi T, Yuki Y, Takahashi H, Sawada S, Mejima M, Kohda T, Harada N, Kong IG, Sato A, Kataoka N, Tokuhara D, Kurokawa S, Takahashi Y, Tsukada H, Kozaki S, Akiyoshi K, Kiyono H. Nanogel antigenic protein-delivery system for adjuvant-free intranasal vaccines. Nat Mater. 2010;9(7):572-578.
- Azegami T, Yuki Y, Nakahashi R, Itoh H, Kiyono H. Nanogel-based nasal vaccines for infectious and lifestyle-related diseases. Mol Immunol. 2018;98:19-24.
- Nakahashi-Ouchida R, Yuki Y, Kiyono H. Development of a nanogel-based nasal vaccine as a novel antigen delivery system. Expert Rev Vaccines. 2017;16(12):1231-1240.
- Nakahashi-Ouchida R, Yuki Y, Kiyono H. Cationic pullulan nanogel as a safe and effective nasal vaccine delivery system for respiratory infectious diseases. Hum Vaccin Immunother. 2018;14(9):2189-2193.
- Yuki Y, Uchida Y, Sawada SI, Nakahashi-Ouchida R, Sugiura K, Mori H, Yamanoue T, Machita T, Honma A, Kurokawa S, Mukerji R, Briles DE, Akiyoshi K, Kiyono H. Characterization and specification of a trivalent protein-based pneumococcal vaccine formulation using an adjuvant-free nanogel nasal delivery system. Mol Pharm. 2021;18(4):1582-1592.
- Fukuyama Y, King JD, Kataoka K, Kobayashi R, Gilbert RS, Hollingshead SK, Briles DE, Fujihashi K. A combination of Flt3 ligand cDNA and CpG oligodeoxynucleotide as nasal adjuvant elicits protective secretory-IgA immunity to Streptococcus pneumoniae in aged mice. J Immunol. 2011;186(4):2454-2461.
- Fukuyama Y, King JD, Kataoka K, Kobayashi R, Gilbert RS, Oishi K, Hollingshead SK, Briles DE, Fujihashi K. Secretory-IgA antibodies play an important role in the immunity to Streptococcus pneumoniae. J Immunol. 2010;185(3):1755-1762.
- Yamamoto M, Briles DE, Yamamoto S, Ohmura M, Kiyono H, McGhee JR. A nontoxic adjuvant for mucosal immunity to pneumococcal surface protein A. J Immunol. 1998;161(8):4115-4121.
- Yamamoto M, McDaniel LS, Kawabata K, Briles DE, Jackson RJ, McGhee JR, Kiyono H. Oral immunization with PspA elicits protective humoral immunity against Streptococcus pneumoniae infection. Infect Immun. 1997;65(2):640-644.
- Kong IG, Sato A, Yuki Y, Nochi T, Takahashi H, Sawada S, Mejima M, Kurokawa S, Okada K, Sato S, Briles DE, Kunisawa J, Inoue Y, Yamamoto M, Akiyoshi K, Kiyono H. Nanogel-based PspA intranasal vaccine prevents invasive disease and nasal colonization by Streptococcus pneumoniae. Infect Immun. 2013;81(5):1625-1634.
- Fukuyama Y, Yuki Y, Katakai Y, Harada N, Takahashi H, Takeda S, Mejima M, Joo S, Kurokawa S, Sawada S, Shibata H, Park EJ, Fujihashi K, Briles DE, Yasutomi Y, Tsukada H, Akiyoshi K, Kiyono H. Nanogel-based pneumococcal surface protein A nasal vaccine induces microRNA-associated Th17 cell responses with neutralizing antibodies against Streptococcus pneumoniae in macaques. Mucosal Immunol. 2015;8(5):1144-1153.
- Yuki Y, Nochi T, Harada N, Katakai Y, Shibata H, Mejima M, Kohda T, Tokuhara D, Kurokawa S, Takahashi Y, Ono F, Kozaki S, Terao K, Tsukada H, Kiyono H. In vivo molecular imaging analysis of a nasal vaccine that induces protective immunity against botulism in nonhuman primates. J Immunol. 2010;185(9):5436-5443.
- Nakahashi-Ouchida R, Uchida Y, Yuki Y, Katakai Y, Yamanoue T, Ogawa H, Munesue Y, Nakano N, Hanari K, Miyazaki T, Saito Y, Umemoto S, Sawada SI, Mukerji R, Briles DE, Yasutomi Y, Akiyoshi K, Kiyono H. A nanogel-based trivalent PspA nasal vaccine protects macaques from intratracheal challenge with pneumococci. Vaccine. 2021;39(25):3353-3364.
- Nakahashi-Ouchida R, Mori H, Yuki Y, Umemoto S, Hirano T, Uchida Y, Machita T, Yamanoue T, Sawada SI, Suzuki M, Fujihashi K, Akiyoshi K, Kurono Y, Kiyono H. Induction of mucosal IgA-mediated protective Iimmunity against nontypeable Haemophilus influenzae infection by a cationic nanogel-based P6 nasal vaccine. Front Immunol. 2022;13:819859.
- Nochi T, Takagi H, Yuki Y, Yang L, Masumura T, Mejima M, Nakanishi U, Matsumura A, Uozumi A, Hiroi T, Morita S, Tanaka K, Takaiwa F, Kiyono H. Rice-based mucosal vaccine as a global strategy for cold-chain- and needle-free vaccination. Proc Natl Acad Sci USA. 2007;104(26):10986-10991.
- Tokuhara D, Yuki Y, Nochi T, Kodama T, Mejima M, Kurokawa S, Takahashi Y, Nanno M, Nakanishi U, Takaiwa F, Honda T, Kiyono H. Secretory IgA-mediated protection against V. cholerae and heat-labile enterotoxin-producing enterotoxigenic Escherichia coli by rice-based vaccine. Proc Natl Acad Sci USA. 2010;107(19):8794-8799.
- Nochi T, Yuki Y, Katakai Y, Shibata H, Tokuhara D, Mejima M, Kurokawa S, Takahashi Y, Nakanishi U, Ono F, Mimuro H, Sasakawa C, Takaiwa F, Terao K, Kiyono H. A rice-based oral cholera vaccine induces macaque-specific systemic neutralizing antibodies but does not influence pre-existing intestinal immunity. J Immunol. 2009;183(10):6538-6544.
- Takeyama N, Yuki Y, Tokuhara D, Oroku K, Mejima M, Kurokawa S, Kuroda M, Kodama T, Nagai S, Ueda S, Kiyono H. Oral rice-based vaccine induces passive and active immunity against enterotoxigenic E. coli-mediated diarrhea in pigs. Vaccine. 2015;33(39):5204-5211.
- Yuki Y, Mejima M, Kurokawa S, Hiroiwa T, Takahashi Y, Tokuhara D, Nochi T, Katakai Y, Kuroda M, Takeyama N, Kashima K, Abe M, Chen Y, Nakanishi U, Masumura T, Takeuchi Y, Kozuka-Hata H, Shibata H, Oyama M, Tanaka K, Kiyono H. Induction of toxin-specific neutralizing immunity by molecularly uniform rice-based oral cholera toxin B subunit vaccine without plant-associated sugar modification. Plant Biotechnol J. 2013;11(7):799-808.
- Yuki Y, Tokuhara D, Nochi T, Yasuda H, Mejima M, Kurokawa S, Takahashi Y, Kataoka N, Nakanishi U, Hagiwara Y, Fujihashi K, Takaiwa F, Kiyono H. Oral MucoRice expressing double-mutant cholera toxin A and B subunits induces toxin-specific neutralising immunity. Vaccine. 2009;27(43):5982-5988.
- Mejima M, Kashima K, Kuroda M, Takeyama N, Kurokawa S, Fukuyama Y, Kiyono H, Itoh K, Mitusi T, Yuki Y. Determination of genomic location and structure of the transgenes in marker-free rice-based cholera vaccine by using whole genome resequencing approach. Plant Cell Tiss Org Cult. 2015;120:35-48.
- Kashima K, Mejima M, Kurokawa S, Kuroda M, Kiyono H, Yuki Y. Comparative whole-genome analyses of selection marker-free rice-based cholera toxin B-subunit vaccine lines and wild-type lines. BMC Genomics. 2015;16(1):48.
- Sasou A, Yuki Y, Honma A, Sugiura K, Kashima K, Kozuka-Hata H, Nojima M, Oyama M, Kurokawa S, Maruyama S, Kuroda M, Tanoue S, Takamatsu N, Fujihashi K, Goto E, Kiyono H. Comparative whole-genome and proteomics analyses of the next seed bank and the original master seed bank of MucoRice-CTB 51A line, a rice-based oral cholera vaccine. BMC Genomics. 2021;22(1):59.
- Kurokawa S, Kuroda M, Mejima M, Nakamura R, Takahashi Y, Sagara H, Takeyama N, Satoh S, Kiyono H, Teshima R, Masumura T, Yuki Y. RNAi-mediated suppression of endogenous storage proteins leads to a change in localization of overexpressed cholera toxin B-subunit and the allergen protein RAG2 in rice seeds. Plant Cell Rep. 2014;33(1):75-87.
- Kurokawa S, Nakamura R, Mejima M, Kozuka-Hata H, Kuroda M, Takeyama N, Oyama M, Satoh S, Kiyono H, Masumura T, Teshima R, Yuki Y. MucoRice-cholera toxin B-subunit, a rice-based oral cholera vaccine, down-regulates the expression of α-amylase/trypsin inhibitor-like protein family as major rice allergens. J Proteome Res. 2013;12(7):3372-3382.
- Kashima K, Yuki Y, Mejima M, Kurokawa S, Suzuki Y, Minakawa S, Takeyama N, Fukuyama Y, Azegami T, Tanimoto T, Kuroda M, Tamura M, Gomi Y, Kiyono H. Good manufacturing practices production of a purification-free oral cholera vaccine expressed in transgenic rice plants. Plant Cell Rep. 2016;35(3):667-679.
- Yuki Y, Nojima M, Hosono O, Tanaka H, Kimura Y, Satoh T, Imoto S, Uematsu S, Kurokawa S, Kashima K, Mejima M, Nakahashi-Ouchida R, Uchida Y, Marui T, Yoshikawa N, Nagamura F, Fujihashi K, Kiyono H. Oral MucoRice-CTB vaccine for safety and microbiota-dependent immunogenicity in humans: a phase 1 randomised trial. Lancet Microbe. 2021;2(9):e429-e440.
- Yuki Y, Nojima M, Kashima K, Sugiura K, Maruyama S, Kurokawa S, Yamanoue T, Nakahashi-Ouchida R, Nakajima H, Hiraizumi T, Kohno H, Goto E, Fujihashi K, Kiyono H. Oral MucoRice-CTB vaccine is safe and immunogenic in healthy US adults. Vaccine. 2022;40(24):3372-3379.
- Tokuhara D, Alvarez B, Mejima M, Hiroiwa T, Takahashi Y, Kurokawa S, Kuroda M, Oyama M, Kozuka-Hata H, Nochi T, Sagara H, Aladin F, Marcotte H, Frenken LG, Iturriza-Gomara M, Kiyono H, Hammarstrom L, Yuki Y. Rice-based oral antibody fragment prophylaxis and therapy against rotavirus infection. J Clin Invest. 2013;123(9):3829-3838.
- Yuki Y, Kurokawa S, Sato S, Sasou A, Matsumoto N, Suzuki A, Sakon N, Goda Y, Takeyama N, Miyoshi T, Marcotte H, Tanaka T, Hammarstrom L, Kiyono H. A heterodimeric antibody fragment for passive immunotherapy against norovirus infection. J Infect Dis. 2020;222(3):470-478.
- Sasou A, Yuki Y, Kurokawa S, Sato S, Goda Y, Uchida M, Matsumoto N, Sagara H, Watanabe Y, Kuroda M, Sakon N, Sugiura K, Nakahashi-Ouchida R, Ushijima H, Fujihashi K, Kiyono H. Development of antibody-fragment-producing rice for neutralization of human norovirus. Front Plant Sci. 2021;12:639953.
- Sato S, Hisaie K, Kurokawa S, Suzuki A, Sakon N, Uchida Y, Yuki Y, Kiyono H. Human norovirus propagation in human induced pluripotent stem cell-derived intestinal epithelial cells. Cell Mol Gastroenterol Hepatol. 2019;7(3):686-688 e685.
- Kiyono H, McGhee JR, Wannemuehler MJ, Frangakis MV, Spalding DM, Michalek SM, Koopman WJ. In vivo immune response to a T-cell-dependent antigen by cultures of disassociated murine Peyer's patch. Proc Natl Acad Sci USA. 1982;79(2):596-600.
- Kiyono H, Cooper MD, Kearney JF, Mosteller LM, Michalek SM, Koopman WJ, McGhee JR. Isotype specificity of helper T cell clones. Peyer's patch Th cells preferentially collaborate with mature IgA B cells for IgA responses. J Exp Med. 1984;159(3):798-811.
- Kiyono H, McGhee JR, Mosteller LM, Eldridge JH, Koopman WJ, Kearney JF, Michalek SM. Murine Peyer's patch T cell clones. Characterization of antigen-specific helper T cells for immunoglobulin A responses. J Exp Med. 1982;156(4):1115-1130.
- Kunisawa J, Gohda M, Hashimoto E, Ishikawa I, Higuchi M, Suzuki Y, Goto Y, Panea C, Ivanov, II, Sumiya R, Aayam L, Wake T, Tajiri S, Kurashima Y, Shikata S, Akira S, Takeda K, Kiyono H. Microbe-dependent CD11b+ IgA+ plasma cells mediate robust early-phase intestinal IgA responses in mice. Nat Commun. 2013;4:1772.
- Beagley KW, Eldridge JH, Lee F, Kiyono H, Everson MP, Koopman WJ, Hirano T, Kishimoto T, McGhee JR. Interleukins and IgA synthesis. Human and murine interleukin 6 induce high rate IgA secretion in IgA-committed B cells. J Exp Med. 1989;169(6):2133-2148.
- Fujihashi K, McGhee JR, Lue C, Beagley KW, Taga T, Hirano T, Kishimoto T, Mestecky J, Kiyono H. Human appendix B cells naturally express receptors for and respond to interleukin 6 with selective IgA1 and IgA2 synthesis. J Clin Invest. 1991;88(1):248-252.
- Xu-Amano J, Beagley KW, Mega J, Fujihashi K, Kiyono H, McGhee JR. Induction of T helper cells and cytokines for mucosal IgA responses. Adv Exp Med Biol. 1992;327:107-117.
- Fujihashi K, McGhee JR, Kweon MN, Cooper MD, Tonegawa S, Takahashi I, Hiroi T, Mestecky J, Kiyono H. γ/δT cell-deficient mice have impaired mucosal immunoglobulin A responses. J Exp Med. 1996;183(4):1929-1935.
- Okahashi N, Yamamoto M, Vancott JL, Chatfield SN, Roberts M, Bluethmann H, Hiroi T, Kiyono H, McGhee JR. Oral immunization of interleukin-4 (IL-4) knockout mice with a recombinant Salmonella strain or cholera toxin reveals that CD4+ Th2 cells producing IL-6 and IL-10 are associated with mucosal immunoglobulin A responses. Infect Immun. 1996;64(5):1516-1525.
- Kiyono H, McGhee JR, Wannemuehler MJ, Michalek SM. Lack of oral tolerance in C3H/HeJ mice. J Exp Med. 1982;155(2):605-610.
- Suzuki I, Kiyono H, Kitamura K, Green DR, McGhee JR. Abrogation of oral tolerance by contrasuppressor T cells suggests the presence of regulatory T-cell networks in the mucosal immune system. Nature. 1986;320(6061):451-454.
- Fujihashi K, Taguchi T, Aicher WK, McGhee JR, Bluestone JA, Eldridge JH, Kiyono H. Immunoregulatory functions for murine intraepithelial lymphocytes: gamma/delta T cell receptor-positive (TCR+) T cells abrogate oral tolerance, while alpha/beta TCR+ T cells provide B cell help. J Exp Med. 1992;175(3):695-707.
- Fujihashi K, McGhee JR, Yamamoto M, Hiroi T, Kiyono H. Role of γδ T cells in the regulation of mucosal IgA response and oral tolerance. Ann N Y Acad Sci. 1996;778:55-63.
- Kweon MN, Fujihashi K, VanCott JL, Higuchi K, Yamamoto M, McGhee JR, Kiyono H. Lack of orally induced systemic unresponsiveness in IFN-gamma knockout mice. J Immunol. 1998;160(4):1687-1693.
- Terahara K, Yoshida M, Igarashi O, Nochi T, Pontes GS, Hase K, Ohno H, Kurokawa S, Mejima M, Takayama N, Yuki Y, Lowe AW, Kiyono H. Comprehensive gene expression profiling of Peyer's patch M cells, villous M-like cells, and intestinal epithelial cells. J Immunol. 2008;180(12):7840-7846.
- Hase K, Kawano K, Nochi T, Pontes GS, Fukuda S, Ebisawa M, Kadokura K, Tobe T, Fujimura Y, Kawano S, Yabashi A, Waguri S, Nakato G, Kimura S, Murakami T, Iimura M, Hamura K, Fukuoka S, Lowe AW, Itoh K, Kiyono H, Ohno H. Uptake through glycoprotein 2 of FimH(+) bacteria by M cells initiates mucosal immune response. Nature. 2009;462(7270):226-230.
- Kishikawa S, Sato S, Kaneto S, Uchino S, Kohsaka S, Nakamura S, Kiyono H. Allograft inflammatory factor 1 is a regulator of transcytosis in M cells. Nat Commun. 2017;8:14509.
- Sato S, Kaneto S, Shibata N, Takahashi Y, Okura H, Yuki Y, Kunisawa J, Kiyono H. Transcription factor Spi-B-dependent and -independent pathways for the development of Peyer's patch M cells. Mucosal Immunol. 2013;6(4):838-846.
- Jang MH, Kweon MN, Iwatani K, Yamamoto M, Terahara K, Sasakawa C, Suzuki T, Nochi T, Yokota Y, Rennert PD, Hiroi T, Tamagawa H, Iijima H, Kunisawa J, Yuki Y, Kiyono H. Intestinal villous M cells: an antigen entry site in the mucosal epithelium. Proc Natl Acad Sci USA. 2004;101(16):6110-6115.
- Kiyono H, McGhee JR, Michalek SM. Lipopolysaccharide regulation of the immune response: comparison of responses to LPS in germfree, Escherichia coli-monoassociated and conventional mice. J Immunol. 1980;124(1):36-41.
- Obata T, Goto Y, Kunisawa J, Sato S, Sakamoto M, Setoyama H, Matsuki T, Nonaka K, Shibata N, Gohda M, Kagiyama Y, Nochi T, Yuki Y, Fukuyama Y, Mukai A, Shinzaki S, Fujihashi K, Sasakawa C, Iijima H, Goto M, Umesaki Y, Benno Y, Kiyono H. Indigenous opportunistic bacteria inhabit mammalian gut-associated lymphoid tissues and share a mucosal antibody-mediated symbiosis. Proc Natl Acad Sci USA. 2010;107(16):7419-7424.
- Fung TC, Bessman NJ, Hepworth MR, Kumar N, Shibata N, Kobuley D, Wang K, Ziegler CGK, Goc J, Shima T, Umesaki Y, Sartor RB, Sullivan KV, Lawley TD, Kunisawa J, Kiyono H, Sonnenberg GF. Lymphoid-tissue-resident commensal bacteria promote members of the IL-10 cytokine family to establish mutualism. Immunity. 2016;44(3):634-646.
- Sonnenberg GF, Monticelli LA, Alenghat T, Fung TC, Hutnick NA, Kunisawa J, Shibata N, Grunberg S, Sinha R, Zahm AM, Tardif MR, Sathaliyawala T, Kubota M, Farber DL, Collman RG, Shaked A, Fouser LA, Weiner DB, Tessier PA, Friedman JR, Kiyono H, Bushman FD, Chang KM, Artis D. Innate lymphoid cells promote anatomical containment of lymphoid-resident commensal bacteria. Science. 2012;336(6086):1321-1325.
- Shibata N, Kunisawa J, Hosomi K, Fujimoto Y, Mizote K, Kitayama N, Shimoyama A, Mimuro H, Sato S, Kishishita N, Ishii KJ, Fukase K, Kiyono H. Lymphoid tissue-resident Alcaligenes LPS induces IgA production without excessive inflammatory responses via weak TLR4 agonist activity. Mucosal Immunol. 2018;11(3):693-702.
- Shimoyama A, Di Lorenzo F, Yamaura H, Mizote K, Palmigiano A, Pither MD, Speciale I, Uto T, Masui S, Sturiale L, Garozzo D, Hosomi K, Shibata N, Kabayama K, Fujimoto Y, Silipo A, Kunisawa J, Kiyono H, Molinaro A, Fukase K. Lipopolysaccharide from gut-associated lymphoid-tissue-resident Alcaligenes faecalis: Complete atructure determination and chemical synthesis of Its Lipid A. Angew Chem Int Ed Engl. 2021;60(18):10023-10031.
- Liu Z, Hosomi K, Shimoyama A, Yoshii K, Sun X, Lan H, Wang Y, Yamaura H, Kenneth D, Saika A, Nagatake T, Kiyono H, Fukase K, Kunisawa J. Chemically synthesized alcaligenes Lipid A as an adjuvant to augment immune responses to Haemophilus Influenzae type B conjugate vaccine. Front Pharmacol. 2021;12:763657.
- Goto Y, Lamichhane A, Kamioka M, Sato S, Honda K, Kunisawa J, Kiyono H. IL-10-producing CD4(+) T cells negatively regulate fucosylation of epithelial cells in the gut. Sci Rep. 2015;5:15918.
- Goto Y, Obata T, Kunisawa J, Sato S, Ivanov, II, Lamichhane A, Takeyama N, Kamioka M, Sakamoto M, Matsuki T, Setoyama H, Imaoka A, Uematsu S, Akira S, Domino SE, Kulig P, Becher B, Renauld JC, Sasakawa C, Umesaki Y, Benno Y, Kiyono H. Innate lymphoid cells regulate intestinal epithelial cell glycosylation. Science. 2014;345(6202):1254009.
- Goto Y, Uematsu S, Kiyono H. Epithelial glycosylation in gut homeostasis and inflammation. Nat Immunol. 2016;17(11):1244-1251.
- Kurashima Y, Amiya T, Fujisawa K, Shibata N, Suzuki Y, Kogure Y, Hashimoto E, Otsuka A, Kabashima K, Sato S, Sato T, Kubo M, Akira S, Miyake K, Kunisawa J, Kiyono H. The enzyme Cyp26b1 mediates inhibition of mast cell activation by fibroblasts to maintain skin-barrier homeostasis. Immunity. 2014;40(4):530-541.
- Kurashima Y, Yamamoto D, Nelson S, Uematsu S, Ernst PB, Nakayama T, Kiyono H. Mucosal mesenchymal cells: Secondary barrier and peripheral Eeducator for the gut immune system. Front Immunol. 2017;8:1787.
- Kurashima Y, Kiyono H. Mucosal ecological network of epithelium and immune cells for gut homeostasis and tissue healing. Annu Rev Immunol. 2017;35:119-147.
- Kamioka M, Goto Y, Nakamura K, Yokoi Y, Sugimoto R, Ohira S, Kurashima Y, Umemoto S, Sato S, Kunisawa J, Takahashi Y, Domino SE, Renauld JC, Nakae S, Iwakura Y, Ernst PB, Ayabe T, Kiyono H. Intestinal commensal microbiota and cytokines regulate Fut2(+) Paneth cells for gut defense. Proc Natl Acad Sci USA. 2022;119(3).
- Kurashima Y, Amiya T, Nochi T, Fujisawa K, Haraguchi T, Iba H, Tsutsui H, Sato S, Nakajima S, Iijima H, Kubo M, Kunisawa J, Kiyono H. Extracellular ATP mediates mast cell-dependent intestinal inflammation through P2X7 purinoceptors. Nat Commun. 2012;3:1034.
- Kurashima Y, Kigoshi T, Murasaki S, Arai F, Shimada K, Seki N, Kim YG, Hase K, Ohno H, Kawano K, Ashida H, Suzuki T, Morimoto M, Saito Y, Sasou A, Goda Y, Yuki Y, Inagaki Y, Iijima H, Suda W, Hattori M, Kiyono H. Pancreatic glycoprotein 2 is a first line of defense for mucosal protection in intestinal inflammation. Nat Commun. 2021;12(1):1067.